
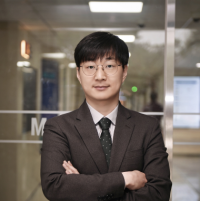
Materials Science and Engineering
Lithium secondary batteries, Computational materials science, AI/robotics-based self-driving laboratoriesResearch contents
Research results
To enhance both the performance and cost efficiency of lithium-ion batteries, the CMDD Lab has developed manganese-based disordered rock-salt (DRX) cathode materials that do not rely on nickel or cobalt. DRX structures diversify lithium-ion diffusion pathways, achieving high energy density while leveraging the affordability and eco-friendliness of manganese. The team successfully optimized manganese content and titanium doping to adjust the heterogeneous nature of DRX structures, leading to the coexistence of spinel, layered, and rock-salt phases. This design improved the reversibility of redox reactions and enhanced cycling stability and energy density. The lab demonstrated a commercial-level energy density of approximately 1050 Wh/kg-cathode with electrodes containing over 96% active material (AM). These groundbreaking results were published as cover articles in Advanced Materials(2023) and Energy & Environmental Science(2024), establishing a sustainable alternative to nickel and cobalt-based cathodes.
We have developed AI-driven non-flammable electrolytes to improve safety and optimize the performance of lithium-ion batteries. Using molecular structure-based AI models, combined with first-principles calculations and molecular dynamics simulations, the lab designed a liquid electrolyte resistant to ignition and thermal runaway. These findings were published as a cover article in Energy & Environmental Science(2023). Additionally, the lab utilized its automated experimental robotics system, ALBATROSS, to generate high-quality electrolyte data around the clock, significantly accelerating development. The AI models predict properties for tens of thousands of solvents and extend predictions to electrolyte formulations, expediting the creation of safer electrolytes for electric vehicles and ESS.
To advance all-solid-state battery technology, the CMDD Lab has developed halide nanocomposite solid electrolytes (HNSEs), ZrO2(-ACl)-A2ZrCl6 (A=Li or Na), using cost-effective materials. First-principles density functional theory (DFT) calculations played a critical role in elucidating the mechanism of interfacial superionic conduction facilitated by oxygen-substituted compounds. Using mechanochemical synthesis with Li2O, the lab improved ionic conductivity for Li+ and Na+ to 1.3 mS/cm and 0.11 mS/cm, respectively, while enhancing compatibility with sulfide solid electrolytes. Furthermore, a fluorinated HNSE, ZrO2−2Li2ZrCl5F, exhibited exceptional high-voltage stability and interfacial compatibility with Li6PS5Cl and cathode materials. The lab successfully demonstrated a lab-scale Li-In||LiNi0.88Co0.11Mn0.01O2 cell with a specific discharge capacity of 115 mAh/g over 2000 cycles. This research underscores the importance of integrating first-principles calculations with innovative synthesis methods to drive advancements in next-generation all-solid-state batteries.
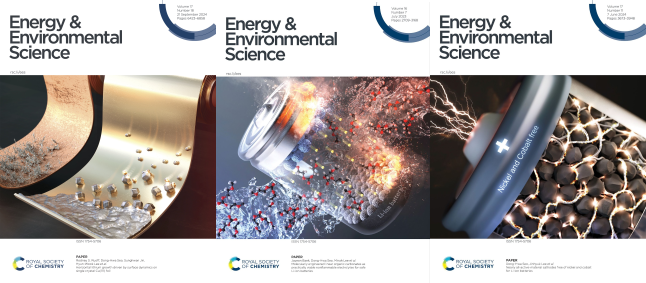
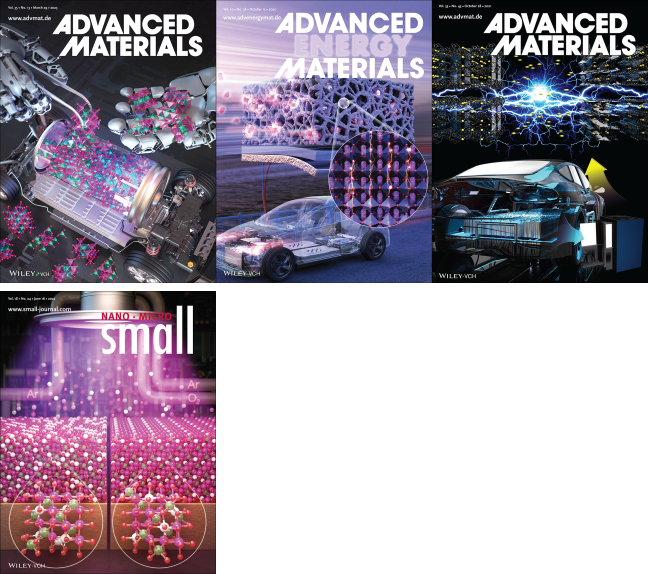